The absence of data on carbon benefits in forest certification schemes necessitates pulling together a number of existing studies and data, and making significant assumptions in order to develop a model. Forest certification systems, which collect significant data on certified forestry operations unfortunately aren’t designed to provide a metric of carbon emissions from forest operations and supply chain activity. Neither FSC nor PEFC (the main forest certification standards) include any requirement for certificate holders to measure the carbon impact of operations, and neither framework has specific international requirements aimed at reducing carbon emissions (see Appendix 1 for details on current difficulties in extending certification data to carbon calculations).
Any carbon accounting requires proxy measures, and there are considerable data limitations and technical barriers to making even the most tentative assessment of certification on tropical forest carbon impacts. To develop our model we compile research on three specific areas of forestry: Long Term Impacts of Felling Cycle, Life Cycle Analysis (which includes felling operations, processing, and transport), and Substitution Benefits (the carbon advantages of sustainable timber over other materials). Of note is the focus only on above-ground carbon stocks. Substantial stocks of carbon are locked in tropical soils as Soil Organic Carbon (SOC), and there is little understanding of the impact of different harvesting operations or conversion events on these stocks (estimates of the total carbon stored as SOC in the tropics vary from less than 200 Pg to 500–650 Pg).[1]
Long Term Impacts of Felling Cycle
Long-term impacts of felling cycle refers to the ability of forest carbon stocks to recover following timber harvesting. RIL (Reduced Impact Logging) and CL (Conventional Logging) operations are the main subject of most studies. For our purposes RIL serves as a proxy for certification. RIL and CL vary in the quantity of biomass extracted (and damaged), and the length of time between subsequent cuttings. How these factors contribute to the long-term health of a forest and its vulnerability to land-use changes is likely to have a significant impact on the carbon emissions associated with supply of tropical timber products.
A review of research reveals mixed results on the question of whether RIL offers real carbon benefits compared to CL. A 2015 meta-analysis notes that modelling studies have implied that the carbon benefits of RIL may be magnified over 40-60 years owing to a reduction in residual damage, but that there is insufficient empirical data to confirm the hypothesis that RIL forests recover more quickly than CL forests.[2]
Other researchers are more confident on the significant long-term carbon storage benefits of RIL in tropical forests. A 2016 analysis by Sasaki et al that modelled long-term impacts of RIL compared to CL posits potentially large carbon benefits of RIL operations. They suggest that the negative consequences of “premature re-entry logging”—common in the lax regulatory environment of tropical regions—lead to the repeated loss of carbon stocks in the affected concession until all marketable trees are harvested.[3]
Sasaki et al created a model based on the assumption that RIL operations log only once at the beginning of the 40-year period, while in CL operations there are additional harvests in years 5 and 20 (see Figures X and Y below). Drawing on a wide range of studies, Sasaki et al estimate an average initial carbon stock in tropical production forest of 174.0 ± 11.6 Mg C ha−1 (± is for confidence interval of 90%) prior to logging, and project that it declines to 121.8 Mg C one year after RIL logging (point P1 in Figure X) but recovers to the pre-logging level or higher by the fortieth year if premature re-entry logging is prevented (point A). In contrast, under CL with two premature re-entries, carbon stocks continue to decline until all merchantable trees are harvested (point D). If premature re-entry logging is allowed, carbon stocks decline by 19.5% and 34.3% for first and second premature logging, respectively.
Sasaki et al estimate that preventing first and second premature re-entry logging across all production forest in tropical regions could reduce emissions by 279.9 and 493.7 Tg C year−1 (1026.4–1810.4 Tg CO2 year−1) respectively, in addition to removing (sequestering) −8.2 Tg C year−1 (−30.1 Tg CO2 year−1) from the atmosphere (Figure Y).
These figures suggest that the emission reductions resulting from reduced impact logging in certified forest operations may be considerable.
In the absence of other research on the likely long-term effects of certified operations on forest carbon stocks at landscape level in the tropics, the Sasaki et al study provides the basis of assumptions in our calculations.
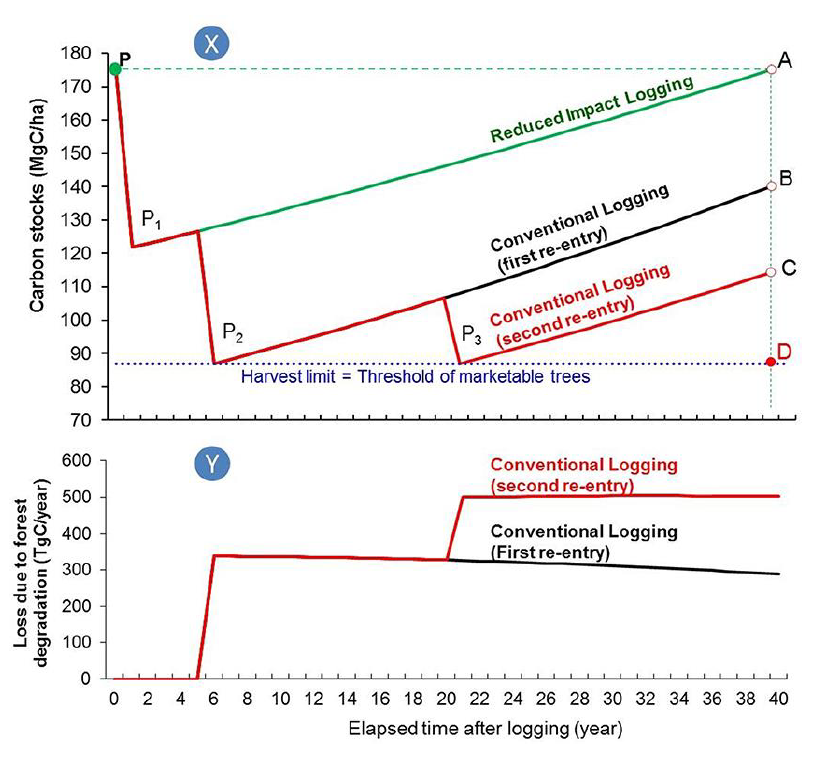
Operational and Lifecycle Costs
Calculating the carbon costs of the physical operations of cutting, transporting, and processing certified tropical timber requires an understanding of a number of processes. Both research on the carbon emissions of RIL and CL forestry practices as well as lifecycle assessments (LCAs) of timber operations can help inform the development of a model for certified tropical timber emissions.
In practice there have been a relatively limited number of comparative assessments of carbon emissions of harvesting operations in certified and uncertified forest. These studies have largely focused on RIL vs. CL with ambiguous results.[4] A meta-analysis of the impacts of tropical selective logging on carbon storage and tree species richness came to the tentative conclusion that “RIL reduces the negative impacts of logging on tree damage but does not support suggestions that RIL reduces loss of aboveground biomass or tree species richness.”[5] It showed that “the volume of wood removed per hectare was by far the best predictor of changes in biomass in response to timber harvest,” suggesting that while RIL results in lower carbon emissions per hectare exploited, RIL and CL lead to the same level of emissions per unit of timber volume extracted. A contradicting study claims that improved logging and skidding practices have the potential to reduce harvesting emissions by 44% (selective logging emits 6% of tropical greenhouse gases annually).[6] Needless to say the data doesn’t clarify reality.
A study in Gabon comparing an FSC-certified concession with an uncertified CL concession on the basis of logging damage, above-ground biomass (AGB), and tree species diversity and composition showed that FSC resulted in less collateral damage per tree cut (averages of 9.1 and 20.9 other trees damaged per tree cut for FSC and CL respectively).[7] Like the meta-analysis, this implied significantly lower carbon emissions on a unit area basis. However, when expressed as impacts per timber volume extracted, the values did not differ between the two treatments.
Another study in East Kalimantan directly comparing the carbon emissions of operations on three FSC certified concessions with those on six non-FSC certified concessions concluded that FSC certified concessions did not have lower overall CO2 emissions from logging activity.[8] However it did find evidence of a range of improved practices using other field metrics. The authors comment that “one explanation of these results may be that FSC criteria and indicators, and associated RIL practices, were not designed to achieve overall emissions reductions. Also, commonly used field metrics are not reliable proxies for overall logging emissions performance. Furthermore, the simple distinction between certified and noncertified concessions does not fully represent the complex history of investments in improved logging practices.” They conclude by suggesting the introduction of a new term called RIL-C that encompasses practices that result in measurable emissions reductions, and call for certification standards to embrace RIL-C requirements.
Assessing the actual emissions associated with the extraction, processing, and distribution of wood products sourced from certified forest operations is typically derived from formal LCA studies (of which carbon footprint is just one aspect). LCAs quantify all material and energy flows and emissions associated with a defined “functional unit” (such as “1 m3 of Malaysian meranti plywood”) at a specified part of its life cycle. LCA data may be made available through stand-alone reports (ideally prepared independently in conformance to ISO standards—ISO14040/44), as verified datasets in large harmonized databases such as Gabi or SimaPro, or as Environmental Product Declarations (EPDs—independently verified and registered documents issued voluntarily by product manufacturers).
A review of the LCAs that might inform the current analysis (see table below) reveals significant limitations. To date there has been no attempt yet to apply a single harmonized LCA methodology to assess the carbon footprint of different wood products on a global or regional scale. There are no formal EPDs in circulation covering any tropical wood product and the GABI database contains no data specific to a tropical hardwood product. LCA reports on tropical wood products are scarce, and those that exist are narrow in scope (limited geographic coverage or few data points). None of the LCA studies related to tropical hardwood products accounted for the impact of tropical timber harvesting on forest carbon pools. LCAs that do consider forestry typically assume that harvesting is carbon neutral over the long term due to regrowth in the forest and only account for direct emissions associated with extraction machinery. They don’t tend to address the question of how to assign carbon debt or credits.
While tropical hardwoods are poorly covered by LCA analysis, there has been comprehensive analysis of American hardwood products as part of a large study by PE International (now Thinkstep) commissioned by the American Hardwood Export Council (AHEC) in 2012. There are strong parallels between the harvesting and production of tropical and American hardwoods—both involve selection harvesting of managed semi-natural forests covering large geographic areas, the transport distances to transport logs from forest to processing location are at least comparable, and the production processes are similar. Our model makes a number of assumptions to translate these LCAs to certified tropical timber.
Table: Summary of carbon footprint results for tropical hardwoods & comparable temperate products
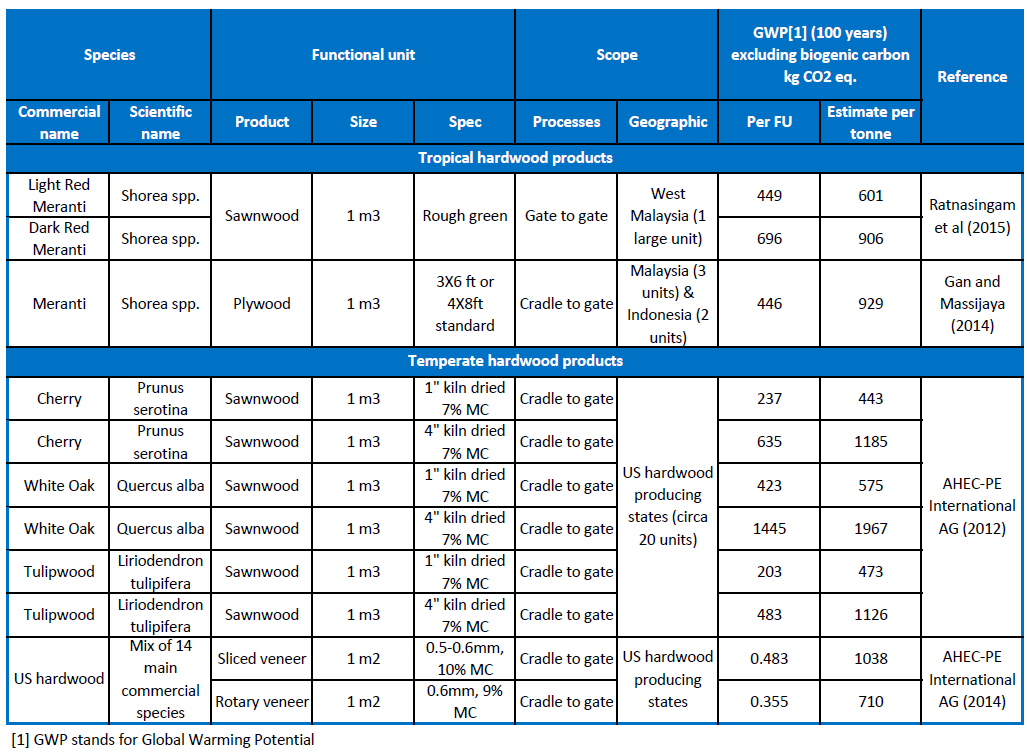
Substitution benefits are situations where certified tropical timber replaces other industrial materials, a proportion of which may require more fossil-fuel energy to produce, be non-renewable, and not offer the carbon sequestration benefits of certified timber. Several studies have attempted to quantify the carbon mitigation benefits of using timber products in place of non-timber products.[10] The most recent, comprehensive, and relevant to the current analysis was undertaken by Leskinen et al for the European Forest Institute in 2018. It notes that the quantification of substitution benefits is not straightforward and involves many uncertainties. To overcome this complexity, Leskinen et al calculate an average “substitution factor” from a meta-analysis of 51 studies comparing the carbon footprint of utilizing wood-based products compared to non-wood products in a wide range of different applications (433 in total). The study indicated that wood products were linked to lower net emissions than substitute products.
Overall, the 51 reviewed studies suggest an average substitution effect of 1.2 kg C / kg C, which means that for each kilogram of C in wood products that substitute non-wood products, there occurs an average emission reduction of approximately 1.2 kg C.” This is more conservative than the substitution effect derived by Sathre & Gustavsson[11] in an earlier 2009 report which draws on a meta-analysis of 20 studies to conclude that in wood product, the displacement factors range from a low of -2.3 to a high of 15.0, with an average of 2.0 (and most lying in the 1.0 to 3.0 range).